Introduction
Since the early 1970s, x-ray computed tomography (CT) has been one of the most versatile, non-invasive investigative techniques in the medical field. It has also enabled nondestructive investigations in many other fields over the past few decades, including industry, archaeology, life science, geoscience, and crime investigations. Unfortunately, conventional x-ray CT systems are only able to achieve spatial resolution at the sub-millimeter scale. Thus, the technique is insufficient for examining the internal structure of objects that require resolution at the micrometer and nanometer scales.
X-ray computed microtomography (μCT), a more technologically advanced technique, overcomes this critical limitation via the use of high-resolution, wide-dynamic- range CCD cameras, high-resolution scintillators, either synchrotron x-ray sources or microfocus x-ray tubes, and software algorithms designed to reconstruct 3D images.
A typical μCT solution comprises an x-ray source, a high-resolution rotating stage with a sample holder, a high-performance scientific CCD camera, and a computer. Depending on the system configuration, either fiberoptic coupling or optical lens coupling is utilized to project an image onto the CCD detection array. Recent advances in all of the above-mentioned technologies enable spatial resolution on the order of microns (see Figure 1).
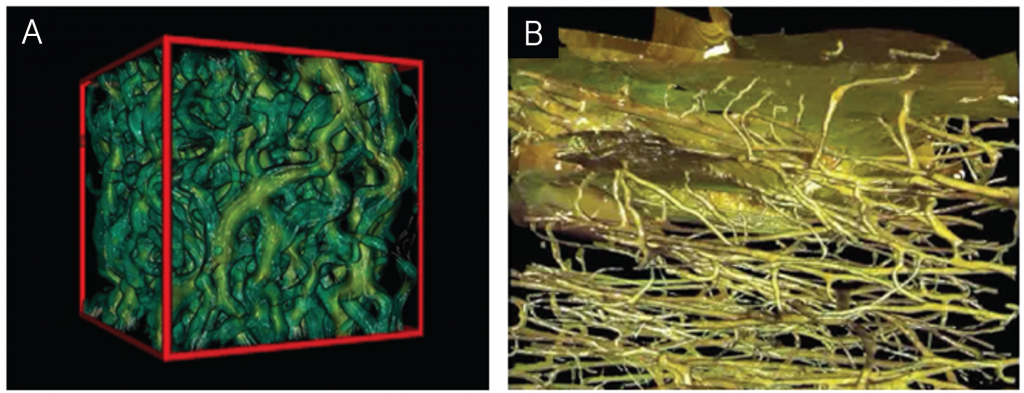
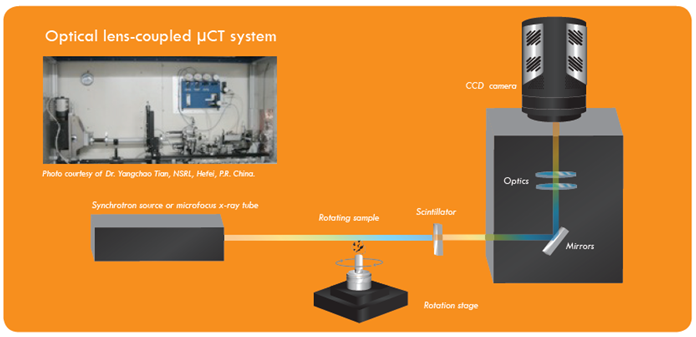
X-ray μCT at a third-generation synchrotron source
Extremely bright (i.e., high-flux) x-ray beams generated by synchrotron sources around the world are steadily increasing the utility of x-ray μCT in a number of research fields. Different configurations of x-ray beams (i.e., high-flux parallel beams or highly focused submicron beams) and high-resolution scintillators make it possible to extend the submicron scale, even below 100 nm, while maintaining reasonable data-acquisition times.
The work of geoscientist Dr. Mark Rivers of the University of Chicago provides an excellent example of the use of an x-ray μCT system at a third-generation synchrotron. In this particular μCT system, the transmitted x-rays are converted to visible light with a single-crystal YAG scintillator, which is imaged via either a microscope objective (5x to 20x) or a zoom lens, with the field of view adjusted between 3 mm and 50 mm. The image is then projected onto a CCD.
Dr. Rivers’ data-collection software uses a layered approach in which each layer has a specific function. Data processing consists of preprocessing, sinogram creation, and tomographic reconstruction (see Figure 3).
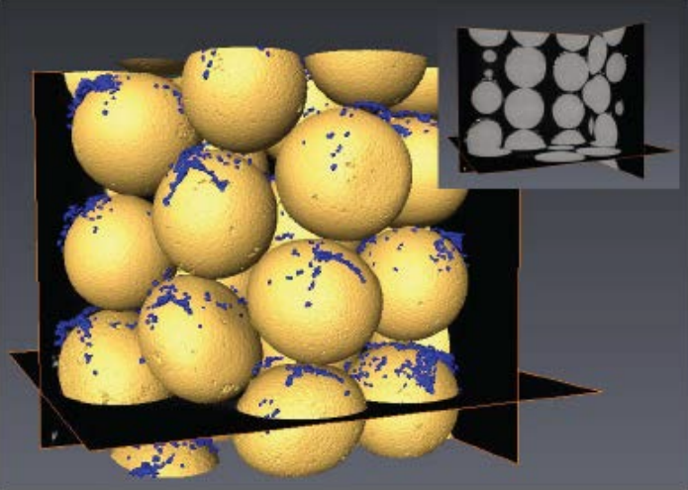
The vast majority of x-ray μCT systems employed at third-generation synchrotron sources feature either a camera based on advanced electron-multiplying CCD technology (e.g., Teledyene Princeton Instruments ProEM) or a shutterless, interline-transfer CCD camera (e.g., Teledyne Photometrics Retiga R6). These types of scientific cameras provide the high speed and outstanding sensitivity required for μCT experiments.
Figure 4A, B and C illustrate the technique’s broad utility for performing work in diverse, complex fields such as materials science.
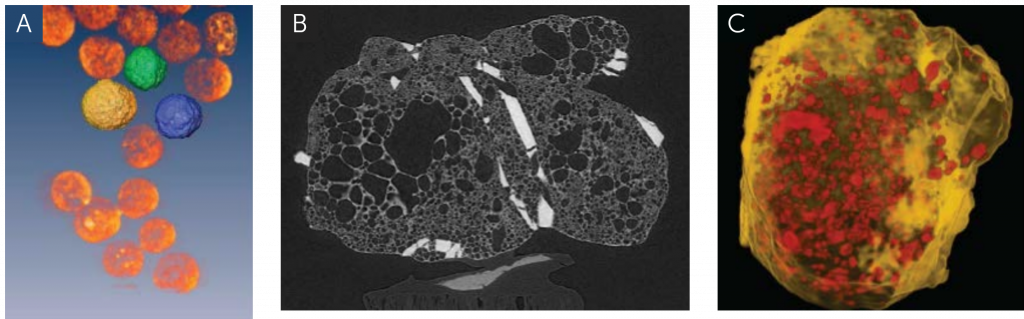
While third-generation synchrotron sources undoubtedly represent an extremely powerful research tool, limited access and expensive operation costs prove prohibitive to many scientists and industries. In the past several years, however, significant advancements in x-ray sources, detectors, sample stages, and computers have led to the design of “desktop” x-ray μCT systems that successfully bring a fair degree of this imaging performance to large and small labs alike.
Desktop x-ray μCT with a microfocus x-ray tube
Instead of relying on an x-ray synchrotron source, desktop μCT systems use a microfocus x-ray tube with a focal spot size of less than 1 μm to achieve spatial resolution of features smaller than 100 nm. This emergent class of desktop systems embodies improvements in x-ray resolution, easy-to-use instrumentation, application flexibility, and affordability.
There are two fundamental kinds of desktop x-ray μCT systems. One uses fiberoptic coupling to project an image onto the CCD, whereas the other utilizes optical lens coupling. If high speed is important in an application (e.g., exposure times of 1 to 10 seconds), then a fiber-optic-coupled system is generally considered advantageous.
To accommodate higher x-ray flux, fiberoptic-coupled desktop μCT systems can use fast-readout, high-sensitivity CCD cameras, such as the Teledyne Princeton Instruments PIXIS-XF.
For x-ray imaging applications that require longer exposure times (e.g., 10 to 30 seconds, or even up to a few minutes), a high-sensitivity detector that can be deeply cooled to reduce dark current is essential. The utilization of Fresnel zone plates to focus the x-rays via diffraction onto the sample allows the use of optical lens coupling in the μCT system, which in turn
necessitates deeper cooling of the detector.
To preserve sensitivity and spatial resolution, optical lens-coupled desktop μCT systems often employ cooled cameras designed with megapixel, high-quantum-efficiency CCDs (e.g., Teledyne Princeton Instruments PIXIS: 1024B/F and 2048B/F)
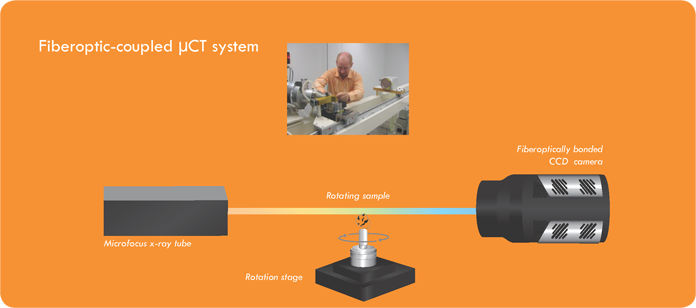
Conclusion
The use of x-ray μCT systems in conjunction with third-generation synchrotron sources has facilitated advances in many research fields (e.g., materials science, geoscience, archaeology, life science, and drug discovery via small-animal CT) by offering truly remarkable performance, but appreciable expenses and tight scheduling bar many from this means of exploration.
Over the past few years, hundreds of new desktop x-ray μCT systems from several manufacturers have been installed in labs around the world. These systems, which rely on microfocus x-ray tubes instead of synchrotron sources, represent an ongoing trend towards miniaturization and personalization of research technology.
Ongoing improvements in x-ray optics, sample stages, and scientific detectors continue to pave the way for new scientific breakthroughs (e.g., the development of electronic packages and more efficient fuel cells). In addition, the intelligent fusion of x-ray phase-contrast imaging and μCT techniques will open still more doors for researchers, enabling the imaging of extremely low absorption materials as well as delivering tools to diagnose cancer at even earlier stages.